Thermoelectrics Background
Heat into electricity; electricity into heating or cooling
Thermoelectrics is a word that sounds familiar largely because it’s regularly misused to describe standard power generation plants. However, since its discovery 200 years ago, it has largely remained a scientific curiousity with limited applications
Put simply, with certain materials, if one side is hotter than the other, electricity is generated – the bigger the temperature differential, the larger the current. And it works in reverse – if you input electricity, a temperature differential is created which can be used to produce either heat or cooling.
Though putting together a thermoelectric device shares some of the same semiconductor processes used in the IT industry, there are in fact far fewer steps involved. To produce usable electricity, p and n-type chips need to be electrically connected in series via metal conductors while being configured thermally in parallel, all sandwiched between a non-electrically conducting hot and cold plates. This type of circuit is known as a thermoelectric module
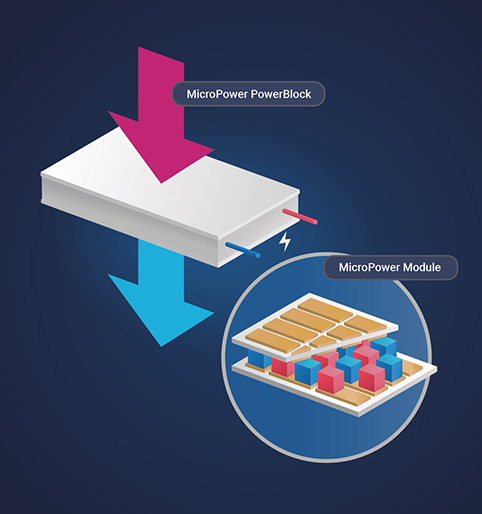
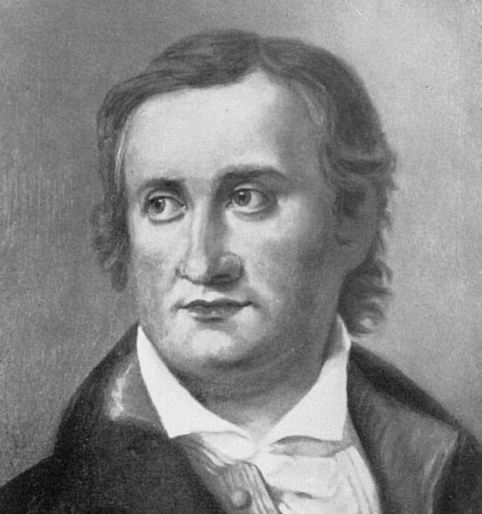
Discovery
The “thermoelectric” effect was actually first discovered nearly two hundred years ago by a German-Estonian scientist called Thomas Seebeck who noticed that a circuit made from two dissimilar metals, with junctions at different temperatures, induced an electrical current. Simple devices using metals in this fashion are still used today, generally to measure temperatures, and are referred to as thermocouples.
A few years after Seebeck’s discovery, a French watchmaker and part-time scientist, Jean Charles Peltier, found that an electrical current would produce heating or cooling at the junction of two dissimilar metals, demonstrating that the thermoelectric effect was thermodynamically reversible.
Semiconductors
Over the remainder of the nineteenth century and early part of the twentieth century, various scientists showed that while metals were sufficient for basic thermocouples, to induce significant amounts of electricity, one would need to use alloys, specifically those with high electrical conductivity (to aid the flow of electrons) and low thermal conductivity (to prevent the heat differential decreasing).
Though interesting, thermoelectrics remained, for the large part, a laboratory curiosity until the emergence of the semiconductor industry in the late 1950s and early 1960s enabled the mass production and analysis of semiconductors for thermoelectric use. While it might seem ridiculous now, there was almost as much excitement at the time around the potential applications of thermal semiconductors as there was for the potential applications of electrical semiconductors – many contemporary observers thought that thermoelectrics would even be able to replace both conventional heat engines, and conventional refrigerators.
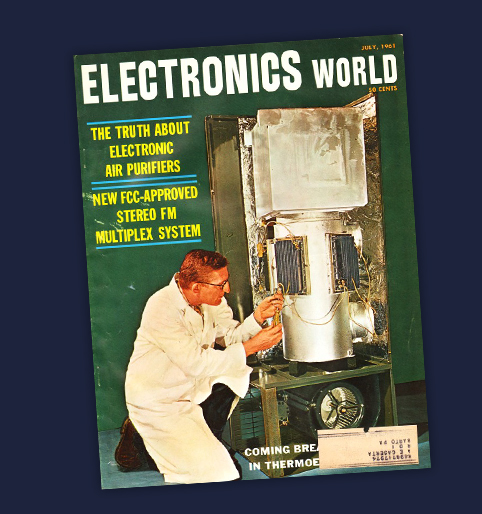
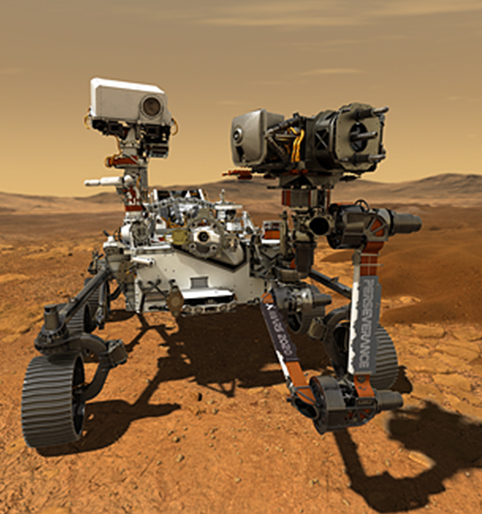
Current Uses
However, efficiencies remained too low, and the field became somewhat of a scientific backwater. At the same time, the best semiconductor minds turned their attention exclusively to electrical semiconductors which led to the now multi-trillion dollar IT industry.
Though conversion efficiencies restricted the use of thermoelectrics for the mass market, niche applications did emerge for situations where reliability or silence were paramount such as optoelectronic cooling, mini refrigerators and remote power generation, the most well-known example of which are the thermoelectric generators used by NASA to power spacecraft and even planetary vehicles, using a decaying nuclear isotope as a heat source, and capable of operating reliably for decades.
Benefits
Scalable
As chips are extremely small, applications can consist of a single chip to an array of hundreds or thousands of chips covering many square meters, depending on the amount of power required, and can be made to cover the unique geometry of the heat source.
High Energy Density
Thermoelectric generators produce significantly more electricity per area, volume and weight than comparable technologies – an order of magnitude more than solar devices for example. Power density is a critical factor in many markets, particularly aerospace and portable power.
Minimal Vibrations or Sound
Due to the fact thermoelectric devices are solid state and therefore have no moving parts, they make little or no noise, and are generally vibration-free.
Durable & Reliable
Also as a consequence of having no moving parts, and not vibrating, thermoelectric generators are generally extremely robust which is why NASA uses them as a source of power in space.
Low Maintenance
Having no moving parts that need replacement is also a significant advantage from a financial perspective and, if the heat source is waste heat, it is essentially free.
Environmentally-friendly
If the heat source is indeed waste heat, the additional electricity produced by the thermoelectric system is essentially emissions-free.
Drawbacks
Low Conversion Efficiency
Literature on thermoelectrics often refers to standard efficiencies of around 10% but, in truth, there are very few commercial devices available today that advertise above 5% and in practice, most do not even perform to that level. How thermoelectric efficiency is measured and to what it specifically refers is currently a topic of considerable debate.
High Cost
Given the relatively low efficiency compared to established technologies, current thermoelectic devices simply do not offer an attractive financial proposition which is why they have been restricted to niche applications where alternatives are not viable.
Moderate Temperatures
By far the most commonly used thermoelectric material is BiTe which can operate up to 250°C. This is fine for cooling applications, which represent most of the current market, but it presents numerous obvious problems when used in a power generation capacity.